When vortex formation limits outflow from a tank, consider a disc-type vortex breaker
Chemical engineers have long said that, while it is easy to get liquid into a tank, it can be difficult to get liquid out. Large line sizes or high-pressure pumps can fill tanks at any desired rate. Tank drainage rates, in contrast, are strictly limited by vortex formation. High-powered pumps cannot increase the drain rate because a vortex extends into the outlet nozzle and blocks the flow. The vortex is caused by the Coriolis effect.
Coriolis forces and the resultant vortex formation are widely misunderstood because they are not well described in chemical engineering textbooks or other information sources. The Wikipedia entry for Coriolis force actually includes a Simpsons TV show episode as a reference. As a result, some explanation is in order.
Coriolis force, like centrifugal force, is sometimes referred to as a “fictitious” or “pseudo” force. This does not mean these forces are in any way unreal. It just means that they derive from changes in our frame of reference, rather than from matter and energy, which give rise to forces like gravitation and electromagnetism. Coriolis force causes a moving object to deflect in the horizontal plane when viewed in a rotating frame of reference (Figure 1).
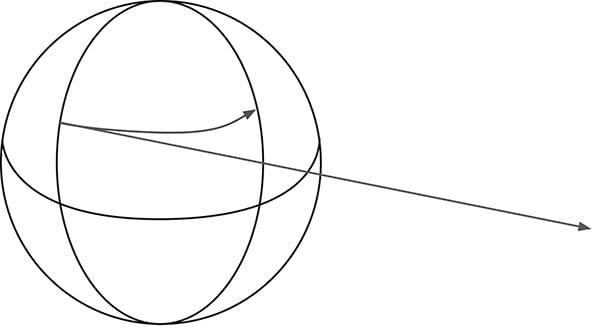
Figure 1. The Coriolis force accounts for the motion of an object within a rotating frame of reference. The purple line shows how a moving object in a non-rotating frame of reference will continue to move in a straight line. The red line shows its path over the surface of the Earth, thanks to the rotation of the Earth.
When liquid drains from a tank, a vertical column of liquid in the center moves down toward the outlet of the tank while the surrounding liquid moves inward horizontally to fill the void. The liquid moving horizontally is subject to Coriolis force, which causes it to rotate. The vortex speeds up because the Coriolis force continues to push the flow faster and away from the center.
Figure 2 shows how the Coriolis force always acts at right angles to the direction of flow, and never points towards the outlet nozzle. Unlike gravity, which is independent of velocity, the Coriolis force increases with velocity. The result is an “acceleration of acceleration”, limited only by fluid viscosity. For water, within half a minute the whole batch is rotating at about one revolution per second.
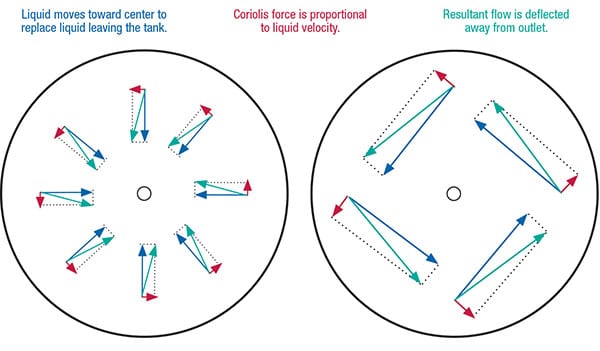
Figure 2: As liquid drains from a tank, the Coriolis force acts at right angles to the flow direction and so sets up a vortex motion
The angular momentum of the fluid is the product of the mass of the fluid, its velocity, and its distance from the center of the tank. Due to the conservation of angular momentum, radius and velocity are inversely related. As the fluid moves inward toward the center outlet, the radius of rotation decreases and so the velocity increases, increasing the rotation rate. Soon, the cone of the vortex extends down to the outlet nozzle and blocks it (Figure 3).
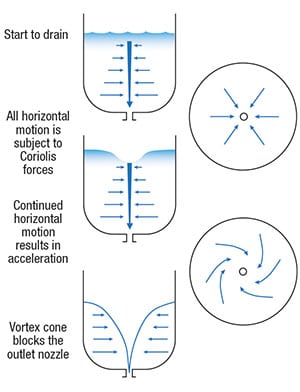
Figure 3: In a tank without a vortex breaker, a vortex will form and quickly grow to the point where it obstructs flow from the bottom outlet
In applications where drain rate is not important, vortex formation is usually not a problem. But there are many applications where the drain rate is important. In those applications, a vortex breaker is required.
Another negative outcome of operating with a vortex is gas entrainment. Gas from above the liquid can be drawn down into the vortex, reducing the capacity of the discharge pump and affecting the performance of processes downstream.
A further problem is reduced cleanability of the tank. Food and pharmaceutical manufacturers have strict requirements for flowrates in clean-in-place (CIP) applications. Typically a spray ball must supply about 3 gal/min per foot of tank circumference (40 L/min per meter) to ensure good cleaning performance. To prevent liquid holdup, which could allow dirt to accumulate, the discharge rate must be at least as large as this. In practice, tanks for CIP must be designed to prevent vortex formation.
Vortex breakers
A vortex breaker is installed to prevent the formation of a vortex when draining a tank. There are two types of vortex breakers: disc-type and cross-type.
Disc type. The disc type (Figure 4) acts as a baffle plate that impedes axial flow without interfering with radial flow. It is typically designed to be three times the diameter of the outlet nozzle and mounted approximately 1 in. (25 mm) above the nozzle. This design eliminates the center vertical column of flow above the disc and allows only horizontal flow in the area below the disc. As the liquid in the bottom of the tank moves horizontally towards the exit nozzle, the liquid above moves downward to replace it. The relatively small volume of liquid in the bottom dish that is moving toward the exit nozzle still experiences Coriolis force, but only for a short time since it is about to leave the tank.
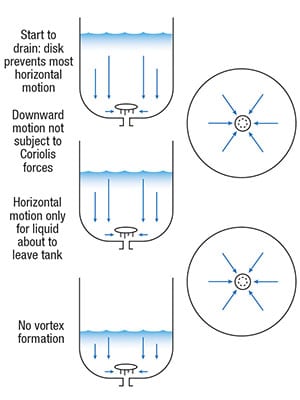
Figure 4. Disc-type vortex breakers work well and do not create undue flow restriction as long as they are suitably positioned
Cross type. The second type of vortex breaker is the cross type (Figure 5). This is supposed to eliminate the formation of a vortex by providing a barrier to rotational flow. In practice, however, small cross-type vortex breakers mounted immediately above the exit nozzle do not work. A little thought shows why: the cross does not influence vortex formation since it impedes rotation only in the immediate vicinity of the outlet, not in the bulk of the tank, which is where the main rotational forces operate.
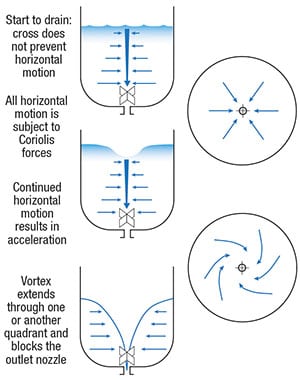
Figure 5. Small cross-type vortex breakers do not work in practice because they have no influence on vortex formation in the main part of the tank
If you watch the draining of a tank without a vortex breaker you will see a vortex form. If the tank has a cross-type vortex breaker you will also see a vortex form. Looking down into a fully developed vortex shows that the cross has no effect whatsoever, with the vortex moving freely from one quadrant to another.
Given the prevalence of vortex formation when draining tanks, it is surprising that cross-type vortex breakers still sometimes appear in engineering designs. One reason may be a fear that the alternative disc-type vortex breaker will present too large a restriction and actually reduce flow out of the tank. This will not occur, however, as long as the flow area under the disc is greater than the area of the outlet nozzle.
In conclusion, the Coriolis effect causes liquid to rotate as it drains from a tank. Unrestricted, the liquid rotation creates a vortex which will block the outlet and limit the drain rate. In cases where a high drain rate is important, such as for CIP or to match discharge pump performance, a vortex breaker is required to prevent liquid holdup and air entrainment. Cross-type vortex breakers are not effective, so the disc type should be installed whenever a vortex breaker is required.■
Edited by Charles Butcher
Authors
Jim Gregory is a process engineer at Fluor Corp. (100 Fluor Daniel Dr., Greenville, SC 29607-2762; Email: [email protected]). He holds a B.A. in biophysics and a B.S.Ch.E. from the University of Connecticut, and an M.Sc. in biochemical engineering from Rutgers University. He has experience in the design and operation of industrial microbiological processes ranging from human-cell-line monoclonal antibodies to diesel fuel.
Katy Lentz is a process engineer at Fluor (100 Fluor Daniel Dr., Greenville, SC 29607-2762; Phone: 864-281-4579; Email: [email protected]). She holds a B.S.Ch.E. from the University of Toledo, Ohio. She has experience in the design and operation of manufacturing and life sciences processes including monoclonal-antibody therapeutics, clean utilities, electrode manufacturing, carbon fiber, and bourbon production.