Supplying steam that meets process needs while protecting steam-system components, such as boilers and steam turbines (Figure 1), against scale, fouling and corrosion, requires purified feedwater (condensate plus makeup water). Depending on the steam pressure required, the specific approach of the water treatment may be shifted to account for a variety of issues. For example, caustic corrosion and phosphate corrosion of boiler tubes are rare in boilers operating below 1,000 psi, but become more of a threat as operating pressures rise above that level. The following outlines water treatment requirements at different steam pressures and some of the important reactions at high- and low-pressure ranges.
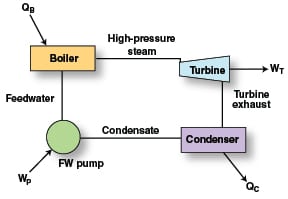
Low-pressure steam systems
At steam pressures below 600 psig (41 bars), boiler feedwater is treated to prevent hard scale formation and corrosion in the boiler. Raw makeup water or heat-exchanger-cooling water from a lake or river typically contains several hundred ppm of cations and anions, most notably calcium, sodium, magnesium, potassium, bicarbonate, chloride, silica, and sulfate, as well as other materials, including suspended solids. As these contaminants enter the boiler, a number of temperature-induced reactions will occur. Two common scale-forming reactions are shown below.
Ca+2 + 2HCO3 –1 > CaCO3(s) + CO2(g) + H2O (1)
Ca+2 (or Mg+2) + SiO3 –2 > CaSiO3 (s) (or MgSiO3 (s) (2)
Even a relatively thin deposit will significantly reduce heat transfer, and a boiler must be fired harder to achieve the same level of steam production. This in turn can lead to overheating of the boiler tubes, which will shorten tube life.
Some type of chemical addition, such as phosphate addition, is used together with gross particulate filtration and decarbonation. For decades, steam-generation chemists have utilized sodium phosphate compounds for corrosion control and prevention of solids deposition in the waterwall circuits of drum-type, steam-generating systems. Alternatives are also available, but can be tricky to control. Boiler water salts are kept from the steam cycle by control of the entrainment carryover and by boiler blowdown.
Medium-pressure steam systems
With steam pressures of 600 to 2,400 psig (41 to 165 bars), control of silica, control of corrosion, and removal of particulate matter are required. Control of silica is necessary to prevent silica from volatilizing with the steam and depositing on the turbine blades. Makeup feedwater demineralization with an anion bed can control the silica levels in the water if it cannot be controlled economically with boiler blowdown. Control of corrosion is mainly done by adding phosphates or using all volatile treatment (AVT). AVT uses ammonia or other volatile amines (morpholine, monoethanolamine) to adjust water pH and control corrosion in that way. Condensate “scavenging” is often used to remove corrosion products from condensate returning from the turbine. Condensate scavenging uses a cation-resin deep bed operated in the sodium or amine form to filter particulate matter and to remove all hardness ions.
High-pressure steam systems
As the boiler pressure increases beyond 2,450 psig (169 bar), demineralization of makeup water of the major contaminant ions, such as sodium and silica, becomes mandatory to satisfy the water quality requirements. Chemical treatment of the boiler or steam-generator system shifts from phosphate treatment to the use of AVT using ammonia or amines to elevate pH and control corrosion in the high-temperature and wet-steam areas of the steam-condensate loop. The optimum pH range depends on the materials of construction; at least 9.3 for all-ferrous systems and 8.8–9.2 for systems containing copper. For high pressure boilers, full-flow condensate polishing is a critical operation for the removal of soluble and insoluble corrosion products, and for the removal of contaminant ions as a result of a condenser in-leakage.
In North America, pressurized-water-reactor (PWR) plants using recirculating-type steam generators (RSGs) have focused their secondary cycle, water-chemistry program on the minimization of insoluble-corrosion-product transport and sodium-to-chloride molar ratio control in the tubesheet crevice areas of the steam generator. A shift to the use of organic amines (monoethanolamine in most cases) for pH control and procedural changes in the resin-regeneration process have been instrumental in achieving the desired improvements in secondary-cycle water chemistry. In addition to AVT chemistry, hydrazine is added to scavenge trace amounts of dissolved oxygen and maintain reducing conditions. Operators must be cognizant, however of the role of oxygen scavengers in flow-accelerated corrosion. Hydrazine reacts with oxygen according to the following equation:
N2H4 + O2 —> 2H2O + N2 (g) (3)
Corrosion
At pressures of at least 1,000 psig or higher, the effect of cooling-water leakages into the system and their effect on rapid corrosion become more pronounced. The reaction shown below is a prime example.
MgCl2 + 2H2O —> Mg(OH)2 (s) + 2HCl (4)
A product of this reaction is hydrochloric acid, which may cause general corrosion by itself, but, when concentrated under deposits, the acid reacts with iron to generate hydrogen. In this mechanism, hydrogen gas molecules, which are very small, penetrate into the metal wall and react with carbon atoms in the steel to generate methane:
2H2 + Fe3C —> 3Fe + CH4 (g) (5)
Formation of the gaseous methane and hydrogen molecules causes cracking in the steel, greatly weakening its strength. Hydrogen damage is very troublesome because it cannot be easily detected.
References
1. Buecker, Brad, CPI Water and Steam Chemistry, Chem. Eng., Feb. 2008, pp. 30–34.
2. Wolfe, Thomas, Boiler-water treatment: At high temperatures, the rules change, Chem. Eng., Oct. 2000, pp. 82–88.
3. Aerts, Peter and Tong, Flora, Strategies for Water Reuse, Chem. Eng. Sept 2009, pp. 34–39.
Editor’s note: Content for this “Facts At Your Fingertips” was taken from the three articles listed in the reference section.