Developments in redox flow batteries are moving at a tremendous pace to meet the growing need for large-scale energy-storage systems, which are used for stabilizing electric power distribution
The global energy-storage market is expected to double, from 1.4 GWh added in 2015 to 2.9 GWh this year, according a report published in July by IHS Markit (London, U.K.; www.ihsmarkit.com). Global grid-connected energy-storage capacity will surge to 21 GWh by 2025, says IHS Markit. Although lithium-ion batteries are expected to dominate the market — accounting for 80% of global installations by 2025, IHS Markit projects — next generation batteries, including advanced redox-flow batteries (RFBs), will also grow in importance.
According to Navigant Research (Boulder, Colo.; www.navigantresearch.com), flow batteries’ market share (in power capacity and revenue) of stationary energy storage across utility, commercial and industrial, and residential sectors, will rise from 145 MW in 2016 ($151 million) to 5.77 GW ($2.92 billion) in 2025. In contrast, Li-ion batteries’ share will grow from 1.06 GW ($789 million) in 2016, to 19.28 GW ($6.36 billion) in 2025. Navigant Research forecasts that new, next-generation flow batteries will begin to penetrate the market in 2019, but vanadium redox will likely remain a significant player within the flow-battery spectrum through 2025, says William Tokash, senior research analyst at Navigant Research. Key drivers for commercial, advanced flow battery technologies to penetrate the market are reduction in costs for raw-materials, manufacturing scale and enhanced safety profiles, says Tokash.
Hoping to tap into this rapidly growing, and important market, extraordinary efforts are underway to bring down the costs of RFBs — the main hurdle in widespread adoption — in order to exploit the advantages this technology offers compared to leading, solid-state Li-ion technology.
In the last 2–3 years, there have been more publications about RFBs than there have been in the previous 30 years combined, says Mike Perry, project leader, Electrochemical Systems, United Technologies Research Center (UTRC; Hartford, Conn.; www.utrc.utc.com). We see a much steeper decline in costs for RFBs compared to Li-ion technology because there are so many areas where improvements can be made, he says. “With RFBs, we are just starting to gather watermelons off the ground, whereas Li-ion technology needs a much higher ladder to ‘pick the low-lying fruit,’” says Perry.
RFB technology today
Redox flow batteries were first invented in Germany in 1949, and the first wave of efforts to commercialize the technology occurred during the U.S. oil crisis of the 1970s.Today, the dominant RFB technology is based on vanadium chemistry (Figure 1). One reason for this is because vanadium is less toxic and easier to handle other multivalent metals, such as chromium.
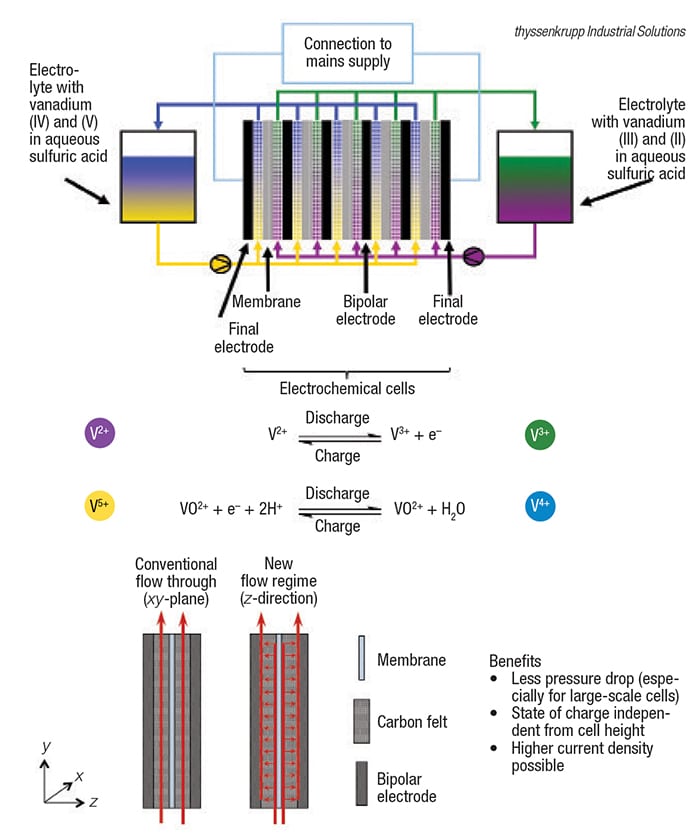
Figure 1. This soon-to-be commercialized all-vanadium RFB technology features a patented flow design to enable scaleup to multimegawatt sizes
According to the U.S. Dept. of Energy’s (DOE; Washington, D.C.; www.energy.gov) Global Energy Storage Database (www.energystorageexchange.org), RFBs account for only 102 of the 1,591 energy-storage projects listed in the database. Of these 102 projects, 59 are vanadium RFBs (VRFBs; 90 MW of power), followed by zinc-bromine (31 projects, 59 MW), iron-chromium (1 project) and others (HBr, zinc-nickel oxide, and zinc iron). Some of the more recent, large-scale VRFB projects are shown in Table 1. One of the largest operating systems, and one of the longest operating VRFBs is a 3-MW system installed at Sumitomo Denseto Office in Osaka Japan, which started up in 2000. This system, which uses 60 50-kW modules, was developed by Sumitomo Electric Industries, Ltd. (Osaka, Japan; www.global-sei.com).
Long life, as demonstrated by the Sumitomo project, is one of the main advantages of RFBs over traditional batteries, such as lead acid and Li-ion. In conventional batteries, the electrodes are continuously degrading over time, explains UTRC’s Perry. In contrast, the electrodes in RFBs are not inherently required to undergo physiochemical changes during the charge/discharge cycles, he says. As a result, RFB manufacturers are able to deliver systems with lifetimes of at least 20–25 yr. In contrast, major components of Li-ion and other batteries need replacing after about 7–10 yr.
RFBs also have the advantage of being able to decouple the power (the rate of electricity flow, in Watts) from the batteries capacity (total amount of energy stored, in Watt-hours), explains, Bill Sproull, vice president of Business Development and Marketing at Energy Storage Systems, Inc. (ESS; Portland, Ore.; www.essinc.com). This feature makes flow batteries much more economical for long-duration energy-storage applications, says Sproull.
UTRC’s Perry agrees. If you want 6 h of discharge, conventional batteries will not work because the electrode required would be too thick, so multiple sets of batteries would be required. With RFBs, you just need to add more chemicals (bigger storage tanks), he says.
Improved designs
Recent RFB breakthroughs have enabled operation with 4–5 times higher current densities than in the past, says Perry, which means one can reduce the size of the membranes required. Based on many years experience with fuel cells, UTRC developed its IDFF (interdigitated flow field) technology, in which the fluids are more evenly and intimately directed across the membranes. The technology is said to generate a much higher power density (2x) compared to traditional flow-through and flow-by designs, according to Vionx Energy Corp. (Woburn, Mass.; www.vionxenergy.com), which is commercializing UTRC’s technology. The company offers a patented battery stack design in its VNX1000 modules based on 1-MW power and 1 MWh building blocks. Vionx is currently installing two 500-kW, 3-MWh systems under DOE-funded projects in Massachusetts, one integrating a photovoltaic (PV) array into the grid in Everett, and a second integrating a wind turbine into the grid in Worcester.
Integration of wind generation with a microgrid is also the goal of the RedoxWind project at the Fraunhofer Institute for Chemical Technology (ICT; Pfinztal, Germany; www.ict.fraunhofer.de). With construction underway (Figure 2), this pilot project, funded by the state of Baden-Wüttemberg and the Federal Ministry of Education and Research, will have a power and storage capacity of 2 MW, 20 MWh when it starts up next year.
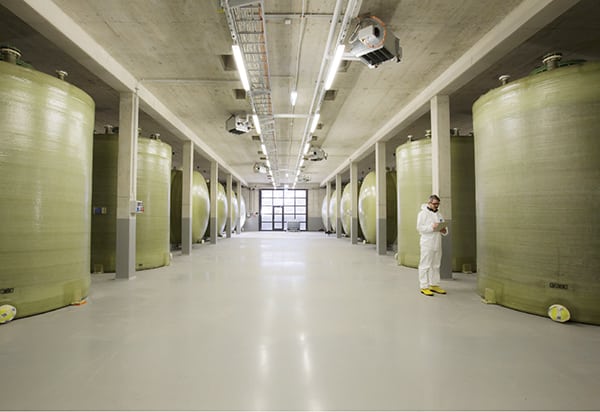
Figure 2. Shown here is the installation of the storage tanks that will hold acidic vanadium solutions for the 3-MW RedoxWind project in Pfinztal, Germany
Meanwhile, thyssenkrupp Industrial Solutions AG (Essen, Germany; www.thyssenkrupp-industrial-solutions.com) is applying its know-how for building chlor-alkali plants in order to take advantage of economy-of-scale for large all-vanadium RFBs (see Chem. Eng., August 2015, p. 9; www.chemengonline.com/commercial-debut-slated-redox-flow-batteries). The company developed a new and unique flow regime. Instead of electrolyte flowing in the xy-plane, as in conventional flow-through designs, the electrolyte is first fed perpendicular to the electrodes ( z direction) through a number of channels (Figure 1, bottom). This feature results in a lower pressure drop, and a state-of-charge (SOC; remaining capacity in a battery) that is independent of the cell height, as well as the ability to operate at higher current densities, says Niels Bredemeyer, senior chemist at the company’s Process Technology business unit.
The patented design is being developed in a pilot system with cell areas of 0.6 m2 at thyssenkrupp’s R&D center in Ennigerloh, Germany. The technology will be commercially available in full size — with cell areas of 2.5 m² — in 2017.
Anticipating the need for larger and affordable components, the companies thyssenkrupp, Centroplast Engineering Plastics GmbH and Eisenhuth GmbH & Co. KG (www.eisenhuth.de), along with two research institutes, launched a joint R&D project in January. The aim of the three-year project — with €3.9-million funding from Germany’s Ministry for Economic Affairs and Energy (BMWi) — is to develop a new, low-cost process to manufacture bipolar plates with surface areas in the square meter range. The new technology will substantially reduce the unit manufacturing costs of RFBs, and is expected to be marketed by thyssenkrupp from 2018.
Meanwhile, Volterion GmbH (Dortmund, Germany: www.volterion.de) has been working — since its formation in 2015 — to commercialize the completely welded stack design developed at the Fraunhofer Institute Umsicht (Oberhausen, Germany; www.umsicht.fraunhofer.de). The design eliminates the problems associated with seals. The company is currently conducting field tests, and plans to introduce compact-size VRFBs for private homes in 2017.
Enhanced chemistries
Because RFBs need pumps, they do not have the same efficiencies as Li-ion batteries. More importantly, the energy density of RFBs is lower, too. To overcome these disadvantages, thereby allowing smaller storage tanks for the electrolytes, improvements are being made by enhanced chemistries. For example, the vanadium technology that Pacific Northwest National Laboratory (PNNL; Richland, Wash.; www.pnnl.gov) introduced in 2011 is said to increase storage capacity by 70%. Three companies have since licensed that technology, including UniEnergy LLC (UET; Mukilteo, Wash. www.uetechnologies.com) and WattJoule Corp. (Lowell, Mass. www.wattjoule.com).
In June, UET announced that its strategic partner Dalian Rongke Power (Dalian, China; www.rongkepower.com) will deploy the world’s largest battery, rated at 800 MWh. The two companies have been working together since 2012 to develop vanadium RFBs. The battery arrays will be made up of ten 20-MW VRFB systems to be installed on the Dalian peninsula, where extreme weather events have caused stress on the electricity grid. When commissioned in 2020, the VRFB battery will be able to peak-shave approximately 8% of Dalian’s expected load.
The battery will be built at Rongke Power’s new GigaFactory, which opens this fall, with phase 1 capacity of 300 MW of VFB electrode stacks, phase 2 capacity of 1 GW, and phase 3 capacity of 3 GW.
Rongke — founded in 2008 by Dalian Bolong Holding Group and the Dalian Institute of Chemical Physics, Chinese academy of Sciences (DICP; www.dicp.cas.cn) — has deployed nearly 30 energy-storage projects, including those for integrating renewable energy, microgrids and isolated power systems. UET was established in 2012, and its founders led the core technology development at PNNL. The company already has almost 20 MW/80 MWh of energy-storage systems deployed, ordered or awarded.
UET has commercialized two products. The Uni.System is packaged in five 20-ft containers, and delivers 600 kW of power with maximum energy of 2.2 MWh. The ReFlex, launched in January, is rated at 100 kW with up to 5-h duration (500 kWh), and is especially suited for commercial and industrial, microgrid and utility applications, says the company. It comes packaged in a single, stackable 20-ft container. Both systems are said to have a 20-year life with no degradation. In July, UET announced that the ReFlex system will be used in a utility grid modernization project in Chattanooga, Tenn., in a project involving the utility EPB and three U.S. national laboratories.
Next-generation chemistries
As efforts continue to improve VRFBs, another R&D front is to find cheaper, more environmentally friendly chemistries than vanadium, which also requires sulfuric acid with the costs associated with corrosion-resistant materials-of-construction and equipment.
For example, ESS Inc. has developed and is producing its All-Iron Flow Battery (IFB) systems. This system (Figure 3) is a hybrid RFB that utilizes a low-cost common electrolyte on both sides of the battery composed of iron, salt and water, explains ESS’s Sproull. During charging, the system electroplates iron to the negative side of the battery and has a redox reaction on the positive side. During discharge, the iron is de-plated back into solution and the chemistry returns to the same ionic state on both sides. The process is repeatable without any degradation in performance over 20,000+ cycles, says Sproull.
ESS has integrated the cells into battery stacks and developed a complete turnkey energy-storage system in 40-ft ISO shipping containers. The power container has the battery stacks, control electronics and a.c.-power conversion system. The energy container has the positive and negative electrolyte tanks and the pump systems. Combined, these two 40-ft containers deliver 100 kW of power and store 8 h of energy (800 kWh).
The primary key feature of the IFB is its low levelized cost of storage (LCOS) over 20–25 year project lifetimes, says Sproull. With low capital, operating and maintenance costs, combined with the ability to cycle multiple times a day without degradation, in an application like smoothing and time-shifting renewable energy, the LCOS of an IFB system can get down to $0.10/kWh, says Sproull. The first commercial system — a 10-kW, 60-kWh unit installed in a microgrid application at Stone Edge Farm (Sonoma, Calif.) — started up in April.
Meanwhile, JenaBatteries GmbH (www.jenabatteries.com) is working to commercialize the all-polymer-based RFB technology that was developed by researchers led by professor Ulrich Schubert at the Friedrich Schiller University (both Jena, Germany; www.uni-jena.de). This water- and polymer-based RFB eliminates the need for vanadium or other metal compounds (which must be very pure), as well as the expensive materials of construction needed for handling the strong acids they are dissolved in, says Schubert. Instead, organic polymers are used as the charge-storage material and an aqueous sodium chloride solution is used instead of sulfuric acid. Because the polymer is a macromolecule, it is much larger than vanadium ions, so an inexpensive cellulose dialysis membrane can be used to separate the cathode from the anode. VRFBs require an ion-selective membrane — typically Nafion — to prevent the metal ions from passing through. This can account for more than 30% of the cost of the reaction cell, says Schubert. The polymer can be made by a standard free-radical polymerization procedure, and is easily upscalable, he says.
JenaBatteries plans to introduce its first series by mid-2017.
Researchers from PNNL have also recently developed an organic aqueous flow battery. When fully developed, the organic RFB is expected to cost 60% less than today’s standard flow batteries, again because the technology enables the used of a simple, inexpensive porous membrane. Storage costs are expected to fall to $189/kWh once the technology is fully developed, says PNNL.
Others are actively looking for new electrolyte materials that are based on organic compounds or organometallic complexes, such as iron with ligands, to eliminate the need for ion-selective membranes. For example, in July, researchers at the John A. Paulson School of Engineering and Applied Sciences, Harvard University (Cambridge, Mass.; www.harvard.edu), published a study that identified a new class of organic molecules, inspired by vitamin B2, that can safely store electricity. The easy-to-synthesize compound (an alloxazine) is highly soluble in alkaline solution. Previously (in 2014), Harvard scientists had developed RFBs that replace metal ions in acidic solutions with quinones, followed by (in 2015) an alkaline RFB based on quinones and ferrocyanide (a common food additive).
New membrane technology
Researchers at the Leibniz Institute for Interactive Materials e.V. (DWI), RWTH Aachen University (Germany;www.dwi.rwth-aachen.de) and Hanyan University (Seoul, South Korea; www.hanyang.ac.kr) have discovered a hydrophobic membrane with nanopores that may be a less expensive alternative to ion-exchange membranes used in vanadium RFBs. These membranes contain tiny (less than 2 nm) water-filled pores and channels that allow protons to quickly pass through, but block the passage of the larger vanadium ions. At all current densities tested (between 1 and 40 mA/cm2), an energy efficiency of 85% was observed, compared to 76% achieved by conventional systems, which suffer from vanadium-ion crossover.
Meanwhile, the Energy Storage Division at DICP has developed porous, non-fluorinated membranes with ultra-high selectivity and stability. When used in a single VRFB cell an energy efficiency of over 90% is achieved at a current density of 80 mA/cm2.