Determining moisture levels in solid materials is important for product quality, handling properties and other considerations. Provided here is an overview of direct and indirect methods for measuring moisture in solids
Water is ubiquitous and is unusually reactive because of its high polarity. It not only bonds strongly to itself and to other polar molecules, but also bonds via physical forces to many other substances. These two factors — abundance and chemical reactivity — make the presence and detection of moisture of great concern to several sectors within the chemical process industries (CPI), such as food, paper and plastics. In these and other industries that produce powders and bulk solids, controlling moisture content can have a profound impact on product quality, as well as on the behavior of the solids in handling operations.
For example, moisture-related microbial growth is a key factor in pharmaceutical dosage forms, and can cause a number of quality issues, such as active pharmaceutical ingredient degradation and disintegration, discoloration or physical hardening of tablets. Similarly, moisture-related microbial growth is a key factor contributing to food spoilage if appropriate precautions for storage are not taken. Acceptable levels of moisture content vary from material to material, but in some cases, even trace amounts of water can adversely affect product quality.
In the context of solid material handling, moisture influences the intermolecular forces between solid particles in at least three ways: (1) it may adsorb onto the solid surface and influence the surface energy; (2) it may alter the surface conductivity and, consequently, the electrostatic charge of particles; and (3) it may condense in the capillary regions contiguous to the true areas of contact. In addition, moisture content provides information about texture, since increasing levels of moisture provides water mobility and lowers the glass-transition temperature.
Aside from product quality and material handling, moisture content can have an impact on shipping costs and product prices, all of which make it a highly consequential measurement. This article provides an overview of how water is taken up in solid materials and reviews both direct and indirect methods of determining moisture levels in solids. The most effective way to select a method is to carefully evaluate the needs of the operation. How the moisture measurement will be used should be a major factor in choosing a method.
Nature of water in solids
Water taken up by solid materials is generally classified as either water that is bound by physical forces or by chemical bonds. Physically bound water includes adsorbed water, trapped or liquid-inclusion water and absorbed water. Physical adsorption of water occurs when water condenses or is held on the surface, in the cracks and crevices of solid materials. Liquid inclusion occurs during the crystallization process, when bubbles of water are trapped. Water absorption is the process of taking up and retaining water uniformly throughout the structure of the host material. Examples of materials that can absorb large amounts of water are silica gel, cellulose, starch and agar.
Chemically bound water, or water of crystallization, is divided into two main categories: bound water and zeolite water. The two are distinguished by what happens to the crystal structure when the water is removed. If the crystal structure changes, it is bound water, but if the original skeletal structure remains, it is zeolite water. Bound water can be further subdivided into several categories, known as coordinated water, lattice water, hydronium-ion water, hydrogen-bonded water and decomposition water.
Mechanism of drying
Drying requires the removal of liquid from the solids. This liquid could be bound moisture or unbound moisture. Unbound moisture normally refers to water on the surface of a solid as a liquid film and is easily evaporated, while bound moisture may be liquid within the solids or trapped in the microstructure of the solid. Bound moisture requires travel to the material’s surface to be evaporated.
Moisture measurement techniques can be classified into direct and indirect methods. Direct methods for the determination of moisture in solids, with the exception of thermal methods, will not distinguish between bound and free moisture in organic materials. Thermal methods have traditionally been used to specify the kinds of moisture in materials. Indirect methods that show promise for distinguishing between bound and free moisture in organic materials are the dynamic dielectric thermal method and microwave infrared (IR), near infrared (NIR), reflectance and nuclear magnetic resonance (NMR) spectrometry.
During drying, two processes occur simultaneously:
1. Heat transfer is the transfer of heat from the surrounding environment to evaporate the surface moisture
2. Mass transfer, the transfer of internal moisture to the surface of the solid, and its eventual evaporation due to heat transfer, is a function of the physical nature of the solid, the temperature and its moisture content.
External drying conditions are important, especially during the initial stages of drying when unbound surface moisture is being removed. Surface evaporation is controlled by the diffusion through a thin film of air in contact with the solid’s surface. The rate at which drying is accomplished is governed by the rate at which the two processes proceed. Energy transfer as heat from the surrounding environment to the wet solid can occur as a result of convection, conduction or radiation and sometimes as a combination of all these. In dielectric, radiofrequency or microwave drying, energy is supplied to generate heat internally within the solid and flows to the external surfaces.
H2O activity and sorption isotherms
Knowing the moisture content of a product alone is insufficient to predict its stability. It is also necessary to know its water activity, a thermodynamic property describing the interactions between water molecules and the matrix (food, for example). Moisture sorption isotherms (the relationship between moisture content and water activity at constant pressure and temperature that describes the sorption process of water molecules into a specific material) are useful when identifying optimal food dehydration and storage conditions. Food manufacturers need to know how long it will be before their product develops mold, gets soggy, goes stale, becomes rancid, cakes, clumps, crystallizes and otherwise becomes unacceptable to the consumer.
A moisture sorption isotherm is a powerful tool for predicting and extending the shelf life of a product. To create a moisture sorption isotherm, a dynamic vapor sorption (DVS) instrument is used. A sample is exposed to a stream of humidity-controlled air, while a microbalance measures tiny changes in weight as the product adsorbs or desorbs water. Once equilibrium is achieved, the instrument dynamically steps to the next preset humidity level. Tests take anywhere from two days to several weeks.
Water activity represents the energy status of the water in the system. It is equal to the relative humidity of the air in equilibrium with a sample in a sealed chamber. It is defined as the vapor pressure of water in a sample divided by the vapor pressure of pure water at the sample temperature.
Water activity is measured using either a capacitance instrument or a chilled-mirror water activity instrument, while moisture content is measured using any one of the 35 different methods listed in the current official methods of AOAC International (Rockville, Md.; www.aoac.org). Water activity and moisture content together provide a complete moisture analysis.
The typical shape of an isotherm reflects the way in which the water binds the system. Weaker water molecule interactions generate a greater water activity. Thus, the product becomes more unstable. Water activity depends on the composition, temperature and physical state of the compounds [1]. Sorption isotherms can be generated from an adsorption process or a desorption process, and the difference between these curves is defined as hysteresis (Figure 1).
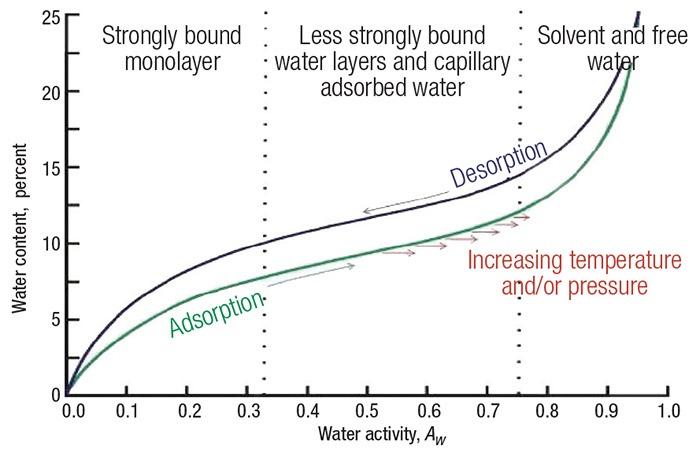
Figure 1. This graph is an example of a sorption isotherm for a typical food product
An isotherm can be typically divided into three regions. The first is strongly bound water, where the enthalpy of vaporization is considerably higher than that of pure water. The bound water includes structural water (H-bonded water) and monolayer water, which is adsorbed by the hydrophilic and polar groups (such as polysaccharides, proteins and so on) of solid products. Bound water is unfreezable and it is not available for chemical reactions or as a plasticizer.
In the second region (less strongly bound), water molecules bind less firmly than in the first zone, and are usually present in small capillaries.
The properties of water in the third region are similar to those of free water that is held in voids, large capillaries and crevices in the solid product. The water in this region loosely binds to solid materials, such as food products [2–5].
Moisture sorption isotherms are extremely important in several areas: modeling the drying process; designing and optimizing drying equipment; predicting shelf-life stability; calculating moisture changes that may occur during storage; and selecting an appropriate packaging material. Understanding of principles and application of moisture sorption isotherms is essential for knowing product-water interactions. Moisture content by water activity is an excellent option for measurement of moisture content, and is especially attractive when both water content and water activity measurements are needed for the same sample. A product-specific isotherm is needed, which can be obtained manually or using an isotherm generator. The precision of this method is the best of any of the secondary methods and exceeds that for loss on drying.
Moisture measurement in solids
The conclusion of a drying process can best be confirmed by measuring residual moisture in the subject product. There are several techniques common within the various CPI sectors to measure the moisture content of solids at the end of the drying step. Moisture-measurement methods can be generally classified as direct or indirect. In the direct methods, moisture is normally removed from the solid material by drying, distillation and other means, and its quantity is found by weighing, titration and so on. On the other hand, for indirect methods, moisture is not removed from the material, but instead, measurements are made of the properties of the wet solid that depend on the amount of water present or the number of hydrogen atoms. Indirect methods must be calibrated with standards that have been prepared by using one of the direct methods. Direct methods generally require manual sampling, several minutes to express a result, and are highly subject to operator error.
The integrity of the material sometimes changes along with loss of moisture as the product is drying. For example, a product might see a chemical change due to decomposition, or oxidation, among other physical changes. There are various techniques to determine the moisture, but a number of factors must be taken into account. These factors include the desired accuracy, the procedure, the sample size and the location of the sample or the probe within a dryer. All have the potential to produce different results.
Direct methods
The following describe direct measurements for moisture in solids.
Vacuum oven or vacuum desiccator. Determination of moisture content of a sample is accomplished by drying in a vacuum chamber. A sample of about 4–5 g is placed in a dry, preweighed glass container and placed in the drying chamber and dried at 102–105˚C. The measurement of the dried sample is carried out at ambient temperature after the sample is cooled. Although simple in concept, there are implicit assumptions and many pitfalls to this approach. First among these is the assumption that water is the only volatile species that will evaporate under the specified drying conditions. Secondly, the conditions of drying (time, temperature, pressure and type of atmosphere) must be carefully specified.
Electrical methods. Another system for moisture detection is based on the fact that many materials change electrical or dielectric characteristics depending on the moisture level in the material. Most of these instruments measure changes in resistance, conductivity or capacitance. Because these techniques are measuring an indirect effect of moisture, calibration is necessary. The calibration is accomplished by comparing the dielectric reading to a known moisture for the sample. A graph is prepared and used to provide the translation of the electrical characteristic to the amount of moisture in the material sample. In automated instruments, these tables are processed by a computer to give readings in percent moisture.
Chemical method. Karl Fischer titration (KFT) is a widely used analytical method for quantifying water content in a variety of products. The fundamental principle behind it is based on the Bunsen reaction between iodine and sulfur dioxide in an aqueous medium. Coulometric Karl Fischer titration is the most widely used technology to determine residual water content. Some chemicals are known to cause problems with Karl Fischer titration, but these chemicals do not usually occur in biologics.
As shown in Equation (1), the Karl Fischer method uses Karl Fischer reagent, which reacts quantitatively and selectively with water, to measure moisture content. Karl Fischer reagent consists of iodine, sulfur dioxide, a base and a solvent, such as alcohol.
I2 + SO2 + 3Base + ROH + H2O —> 2Base + HI + Base + HSO4R (1)
The alcohol (ROH) reacts with sulfur dioxide (SO2) and base to form an intermediate alkyl sulfite salt, which is then oxidized by iodine to an alkyl sulfate salt. This oxidation reaction consumes water. Water and iodine are consumed in a 1:1 ratio in the reaction. Once all of the water present is consumed, the presence of excess iodine is detected voltametrically by the titrator’s indicator electrode. In other words, information about an analyte is obtained by measuring the current as the potential is varied. That signals the endpoint of the titration. The amount of water present in the sample is calculated based on the concentration of iodine in the Karl Fisher titrating reagent (titer) and the amount of Karl Fisher reagent consumed in the titration.
There are two types of Karl Fischer reactions: volumetric KFT and coulometric KFT. In volumetric KFT, iodine is added mechanically to a solvent containing the sample via the burette during the titration. Water is quantified on the basis of the volume of Karl Fischer reagent consumed. Volumetry is best suited for the determination of water content in the range of 100 parts per million (ppm) to 100%. In coulometric KFT, iodine is generated electrochemically in situ during the titration. Water is quantified on the basis of the total charge passed (Q), as measured by current (amperes) and time (seconds), according to the relationship in Equation (2).
Q = 1 C (Coulomb) = 1 A × 1 s, where 1 mg H2O = 10.72 C (2)
Coulometry is best suited for the determination of water content in the range of 1 ppm to 5%.
Capacitive method. This is the least expensive and easiest-to-handle moisture-measurement method, and it provides accurate, repeatable results for a variety of materials at high frequencies. The capacitive (also called high-frequency dielectric shift) sensor measures moisture based on the difference between the dielectric constants of water and the material located between the sensor’s capacitor electrodes. Most solids have a dielectric constant between about 4 and 13. Water has a dielectric constant of about 80. The sensor measures this difference to determine the material’s percentage of moisture content by weight. When the capacitive sensor measures an increase in the material’s capacitance toward that of water’s dielectric constant, the increase indicates the material contains more moisture. The capacitive sensor provides realtime measurement and can be used as either a contact or noncontact method.
Thermogravimetric (loss on drying) method. A loss-on-drying (LOD) instrument for bulk-solid moisture analysis uses the same theory as standard oven methods: An empty sample pan is weighed and tared. A sample is added and recorded as the initial starting weight. Heat is applied to the sample to evolve moisture (or other volatile compounds). The difference in sample weight is recorded and calculated as percent moisture using the following relationship: Initial weight of the sample – Final weight of the sample = Loss in weight; Loss in weight × 100 = % moisture.
With a LOD instrument, the balance and heat source are coupled together, allowing the user to view realtime moisture curves and rate graphs as moisture is evolved. In the integrated system, test times are substantially faster with the same level of accuracy one would expect from traditional oven methods. The use of thermogravimetric analysis, with evolved gas mass spectrometric monitoring, was a successful alternative for moisture analysis in lyophilized materials [6]. The authors reported that the thermogravimetric analysis (TGA) method was capable of analyzing these samples without any fundamental difficulties, other than the need to pack sufficient material into the TGA sample pan. By contrast, these lyophilized samples could not be successfully analyzed by the coulometric Karl Fischer method without costly repeated changing of the reagents and cleaning of the cell every 2–3 samples.
Moisture analyzer HE53, made by Mettler Toledo (Figure 2) is a very common instrument using a thermogravimetric principle. Vapor Pro XL, made by AMETEK Brookfield (Figure 3) is also a thermogravimetric base unit ideal for nearly any application in which Karl Fischer titration is used. The SMART 6, from CEM (Figure 4), is a microwave moisture analyzer for rapid moisture measurement.
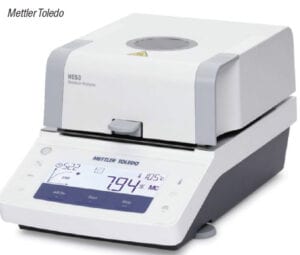
Figure 2. An example of a thermogravimetric moisture analyzer is shown here
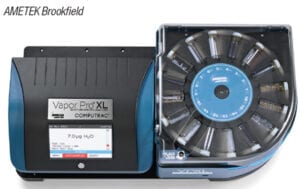
Figure 3. This thermogravimetric device is ideal in applications where Karl Fischer titration is used
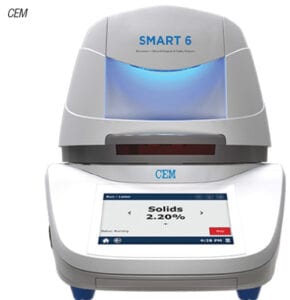
Figure 4. Shown here is an example of a microwave-based moisture analyzer device
Indirect methods
For moisture determination instruments in general, applying the concepts of reflection and absorption of electromagnetic energy is becoming widespread, particularly for in-line or on-line measurements. These techniques are based on the finding that water (as well as other chemicals) has very specific absorption wavelengths. Instruments based on the absorption of near infrared (NIR) and microwave radiation technology are the most common. Although the two techniques are different, both are based on the absorption of electromagnetic energy. When the moisture content of a calibration sample is known, the absorption results can be referenced to the moisture content of the sample. The use of lasers for this purpose is a newer, but related, technique.
Near infrared (NIR). Infrared sensors measure the moisture content by passing a light beam at infrared wavelengths through the material and measuring the ratio between absorbed and reflected wavelengths. NIR spectroscopy is a well-established technology for online moisture testing. NIR reflectance spectrometry utilizes the near-infrared diffuse reflectance radiation from a solid sample at several discrete wavelengths to predict moisture and other chemical species in the material. A light source is collimated and filtered into specific wavelengths. Water displays the characteristic absorption at a near-infrared range between 1,100 and 2,450 nm. The filters are chosen such that one wavelength will be absorbed by water (sample beam) and one wavelength will be unaffected by water (reference beam). In consequence, the amplitude ratio of the two reflected wavelengths will be proportional to the amount of water in the product. This filtered beam is directed onto the surface of the sample to be measured. One part of the light is reflected back to a detector. Chemometric methods then also allow the calculation of water contents in different matrices. NIR can be universally applicable with any molecule containing C-H, N-H, S-H, or O-H bonds. It does not require sample preparation and is very fast.
Handheld portable NIR units (Figure 5) are offered by the industry that allows even less-trained personnel to take portable, instant moisture readings of powdered bulk products as needed. The approach involves moisture meters that utilize NIR light, a highly accurate, non-contact secondary measurement method that can deliver immediate, laboratory-quality moisture readings. In addition, because the process is non-destructive, samples remain unaltered so they can be used for additional tests or put back into the product stream. NIR is limited in the fact that it only measures surface moisture and does not penetrate the interior of the material.
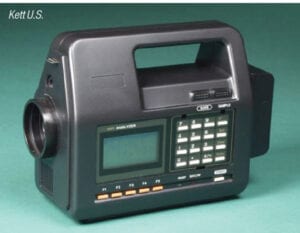
Figure 5. Handheld near infrared (NIR) moisture analyzers allow personnel to take moisture readings with a portable instrument
Microwave moisture measurement. Microwaves are a part of the electromagnetic spectrum, with wavelengths in the centimeter range, bounded by the longer radio waves and the shorter infrared waves. The frequencies of microwaves range roughly between 10 and 10,000 MHz. The microwave oven is a device in which dielectric heating is affected by high-frequency electromagnetic waves. This type of heat forms as a result of dielectric losses that occur in a material that is located between the metal walls of the oven, which act as a capacitor connected to a high-frequency generator, the magnetron. The effectiveness of dielectric heating in a microwave oven is strongly dependent on the polarity of the material exposed to the electromagnetic field. Polar molecules (dipoles), in which the centers of positive and negative charge do not coincide, are in thermal equilibrium in the absence of an electromagnetic field. When an electromagnetic field is applied, the dipoles orient themselves quickly and repeatedly in the direction of the field. The continual molecular motion generated by the alternations of the field causes the material to heat by intermolecular friction. This type of heating is rapid because, unlike conventional heating, the heat does not need to be conducted through the material starting from the surface but is generated rather uniformly inside the material.
Microwave sensors measure the loss of energy of microwaves passed through the sample at the point of emission, and use the difference in their speeds in different media to calculate the moisture content. This loss in energy increases with the amount of water that the medium contains, with the result that, as the water concentration increases, less energy will reach the other side of the medium. Microwave sensors are very accurate in measuring moisture in very fine uniformly distributed materials. Microwave techniques are attractive because at these frequencies (below the 2.5 GHz range} the electrical energy is strongly absorbed by water because of the dipolar character of water molecules, and the effect of ionic conductivity is negligible. Moisture content has been related to dielectric properties. The advantage of using this technique over the traditional oven-dried methods is that permittivity can be measured in near realtime. Because of its high susceptibility to water, microwave technology has established a strong foothold for moisture analysis of food and agricultural products.
In the pharmaceutical industry, the response to the process analytical technology (PAT) initiative of the U.S. Food and Drug Administration has been a rising interest in the development of microwave sensors for the remote, on-line moisture sensing of pharmaceutical intermediates and products during processing. Compared to NIR waves, which are sensitive primarily to surface moisture, the lower frequencies and thus higher penetration depths of microwaves are more advantageous because they allow the detection and quantification of moisture entrapped or embedded within a product [7]. In two recent studies, microwave sensors were used for in-situ moisture determination of wetted powders and granules during high shear granulation [8] and fluidized-bed drying [7], respectively.
The use of a microwave moisture meter, as opposed to a humidity sensor, provides a very consistent and high product quality while reducing operating and maintenance costs. When connected to a controller or a process control system, the operator has the opportunity to interact with the measurement results in real time. For online moisture measurement in production processes, the microwave sensor (Figure 6) is valuable in many process stages: in the refining of wood pulp, during resin treatment, during pressing/dewatering, and during the final drying. When used for measuring the moisture content of sawdust or larger wood chips, the microwave sensor provides exceptionally accurate measured values if the wood pulp is homogeneous, well mixed, and pre-dried to avoid “moisture nests” in the wood pulp mixture.
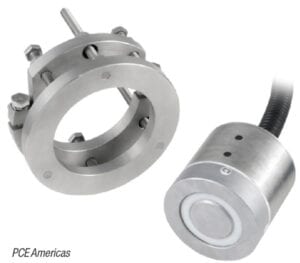
Figure 6. Microwave moisture sensors, like the one shown here, can be valuable in many different process stages
Nearly all bulk goods can be measured by microwaves. There are very few materials with restrictions. Problems can, however, arise in the rare case where the water molecules are not able to rotate, for example, if they are captured in capillaries. For the same reason, crystal water and ice do not respond to the measurement. To address fluctuation in the drying of grain continuously researchers have used online measurement using the microwave resonator technique [9].
Nuclear magnetic resonance (NMR). The low-resolution broad-banded NMR spectrum of water and ice shows that the tightly bound protons in the solid and the mobile water protons in the liquid can be separately quantified. This approach can also be used to determine the amounts of free and bound moisture in solid substances. [10, 11]. In a 2002 paper [12], researchers at the Lawrence Berkeley National Laboratory (www.lbl.gov) developed a device that measures the water content of woodchips, pulp and brown stock for the paper industry. This device employs a permanent magnet as the central part of an NMR measurement system. The report describes the magnet and the NMR measurement system. The results of water content measurements in woodchips in a magnetic field of 0.47 T are presented. They also found, as was reported in an earlier study, that the NMR moisture content measurements can differentiate between pore moisture and surface moisture. Moisture in the form of liquid water can be differentiated from water in the form of ice. Being able to differentiate water from ice means that NMR can be used in a number of areas in the forest products industry.
Optical method. Because the water molecule has distinct optical properties, moisture detection using optical techniques allows a new method of on-line measurement. This approach is non-intrusive to the product, capable of realtime measurement and can be automatic. The optical absorption of water occurs at distinct wavelengths, with very high absorption in the IR range and a slightly reduced absorption in the NIR. Depending on the moisture range of interest and the substance in which it is being measured, each wavelength region can be utilized with the same net benefits. Typical IR measurement are better for moisture levels less than ~1%, while NIR measurement is better for moisture greater than ~1%. Sampling options range from flow cells with selectable path lengths, to measuring heads that mate to sight glasses in vessels, to fiber probes for contact or non-contact measurement in various insertion points. The dynamic-equilibrium moisture content of rice by the optical method was measured and was consistent with the actual drying method. The experimental results were compared with the results of other measurement methods for correction, in order to achieve real-time measurement and analysis of the batch re-circulating rice drying process [14].
Edited by Scott Jenkins
References
1. Fabra, MJ, Talens P, Moraga G, Martínez-Navarrete N. Sorption isotherm and state diagram of grapefruit as a tool to improve product processing and stability, J. Food Eng., July 93 (1), pp. 52–58, 2009.
2. Fortes, M., Okos, MR. Drying theories: Their bases and limitations as applied to foods and grains. Found in Mujumdar AS (Ed.). “Advances in Drying, vol. 1,” Hemisphere Publishing Corporation, Washington, D.C., p. 301, 1980.
3. Kinsella, JE, Fox, PF. Water sorption by proteins: Milk and whey proteins. Crit. Rev. Food Sci. Nutr. April–Jun; 24 (2), pp. 91–139, 1986
4. Mohsenin, NN. “Physical properties of plant and animal materials, 2nd ed.” Gordon and Breach, New York, N.Y., p. 62, 1986.
5. Van den Berg, C., Bruin, S. Water activity and its estimation in food systems: theoretical aspects, article found in Rockland, LB and Stewart, GF (eds.). “Water Activity: Influences on Food Quality,” Academic Press, New York, N.Y., p. 236, 1981.
6. Matejtschuk, P., and others, Use of Thermogravimetric Analysis for Moisture Determination in Difficult Lyophilized Biological Samples, American Journal of Analytical Chemistry, vol. 7, pp. 260–265, 2016 (Published Online March 2016 in SciRes.).
7. Buschmüller, C., Wiedey, W., Döscher, C., Dressler, J., Breitkreutz, J., In-line monitoring of granule moisture in fluidized–bed dryers using microwave resonance technology. Eur. J. Pharm. Biopharm., 69, pp. 380–387, 2007.
8. Gradinarsky, L., Brage, H., Lagerholm, B., Björn, I.N., Folestad, S., 2006. In-situ monitoring and control of moisture content in pharmaceutical powder processes using an open-ended coaxial probe. Meas. Sci. Technol., 17, 1,847–1,853, 2006.
9. Kocsis, L., Schlemm, U., Richter, H., Mellmann, J., Farkas, I., Online Microwave Measurement of the Moisture Content of Wheat, “Proceedings of the 17th World Congress,” The International Federation of Automatic Control, Seoul, South Korea, July 6–11, pp. 631–635, 2008.
10. J. Mitchell, Jr. and D. M. Smith, “Aquametry,” Wiley, New York, Part I, 1977; Part II, 1980; Part III, 1984.
11. Ward, R.L., Lawrence Livermore National Laboratory, Livermore, Calif., private communication, September 1982.
12. Barale, P.J., Fong, C.G., Green, M.A., and others, The Use of a Permanent Magnet for Water Content Measurements of Wood Chips, IEEE Transactions on Applied Superconductivity, vol. 12 (1), pp. 975–978, March 2002.
13. Zambrano, M. and others, Assessment of moisture measurement methods of dried food products in small scale operations in developing countries: A review, Trends in Food Science and Technology, 88, pp. 484–496, 2019.
14. Hung, JungShei, Chern-Sheng, Lin, An optical automatic measurement method for the moisture content of rough rice using image processing techniques, Computers and Electronics in Agriculture, vol. 85, pp. 134–139, July 2012 (https://doi.org/10.1016/j.compag.2012.04.012).
Author
Dilip M. Parikh is president of the pharmaceutical technology development and consulting group DPharma Group Inc. (Ellicott City, MD 21042; Email: [email protected]). As an industrial pharmacist, Parikh has more than 35 years of experience in product development, manufacturing, plant operations and process engineering at various major pharmaceutical companies in Canada and the U.S. Prior to staring DPharma Group, he held the position of vice president of operations and technology at Synthon Pharmaceuticals in North Carolina and vice president and general manager at Atlantic Pharmaceuticals Services in Maryland. Parikh is the author of the recently published book “How to Optimize Fluid-bed Processing Technologies.” He is also the editor of “Handbook of Pharmaceutical Granulation” 3rd ed. He has authored several book chapters and articles on various pharmaceutical technologies, including quality by design, process assessment and contract manufacturing. He has been an invited speaker at scientific conferences worldwide on solid-dosage technologies development and manufacturing.